Rhythm of the night: how circadian clocks influence nocturnal creatures
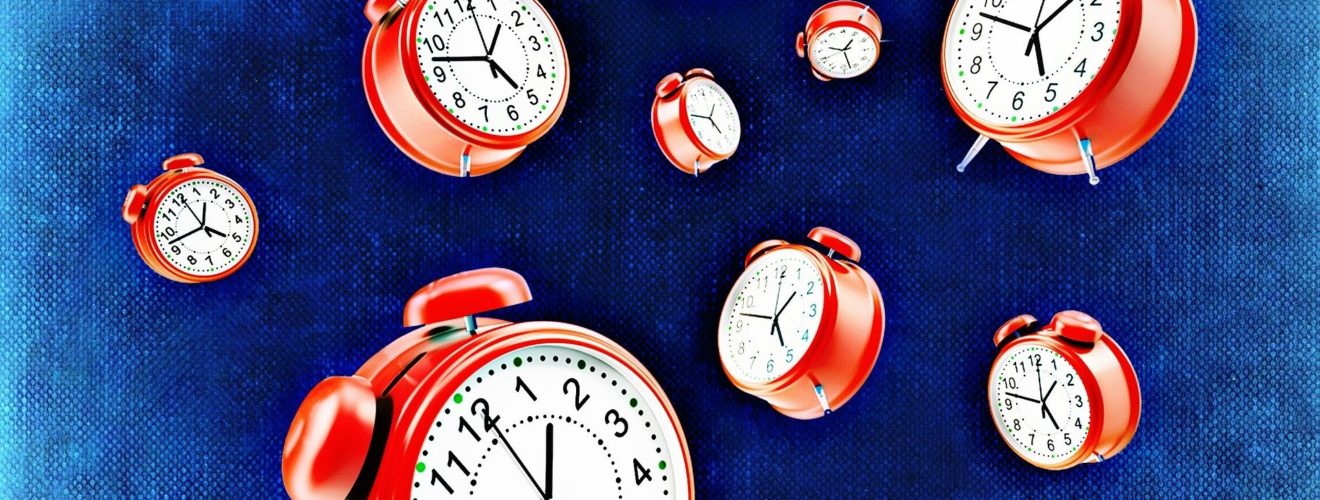
You may be reading this article over your morning coffee, or at midnight – nourishing your brain with scientific tidbits before drifting into a deep slumber. Whatever time you have chosen to peruse, it will be at some point during your natural sleep/wake cycle: your circadian rhythm. Since the award of the Nobel Prize in Physiology or Medicine to Hall, Rosbach, and Young for their work on circadian rhythms in 2017 1, we have all heard this euphonious term more frequently, but how do the factors that regulate sleep/wake cycles differ between nocturnal (active at night) and diurnal (active during the day) animals? Most living things adhere to a cyclic pattern of activity over a period of time; humans generally follow a 24-hour circadian rhythm, incorporating around eight hours of sleep (usually at night) and 16 hours of wakefulness. This is because our circadian rhythm is partially regulated by light exposure, which in turn dictates the activity of genes and hormones related to our internal biological clocks. Some mammals adopt completely nocturnal lifestyles and even hibernate or undergo periods of dormancy. To find the conductors of these natural rhythms, we need to get cellular…
SCN: If you say it loud enough you’ll always sound precocious!
In mammals, biological clocks are physical structures present in most organs and tissues in the body; they are made of proteins that interact with other molecules and change their activity throughout the day depending on environmental factors. Each of these biological clocks is connected to a ‘master clock’ located in the hypothalamus region of the brain – our very own version of the Greenwich Time Ball! This master clock consists of thousands of neurons and astrocytes (a type of star-shaped cell in the central nervous system that supports neurons), together forming a structure known as the suprachiasmatic nucleus (SCN). Crucially, the hypothalamus is also connected to our optic nerve. This means that our sensitivity to light and changes in our experience of light have direct effects on the SCN.

When the light begins to change, I sometimes feel a little strange…
Studies into the synchronisation of animal biological clocks to the solar clock2 have shown that light levels affect the electrical excitation, or the spontaneous firing rate (SFT), of neuron cells in the SCN. Increases in light result in a momentary change in electrical charge (a depolarisation, in this instance) of core SCN neurons connected to the optic region of the hypothalamus. This sudden change is then amplified as the charge passes from one cell to the next, causing an overall increase in spontaneous firing rate (the pulse of the electrical signal passes from one cell to the next, much like a Mexican wave). A state of depolarisation in SCN cells is associated with daylight hours whereas hyperpolarisation is associated with hours of darkness – this is the same in all mammalian SCNs, regardless of nocturnal or diurnal behaviour. Increases in light (at dawn) and decreases (at dusk) act as the entraining indicator that tells our body when the phase of the day is changing, keeping our biological clock in time with that of the natural world. Why is it then that for nocturnal mammals, a reduction in light is in fact the trigger for waking and activity?
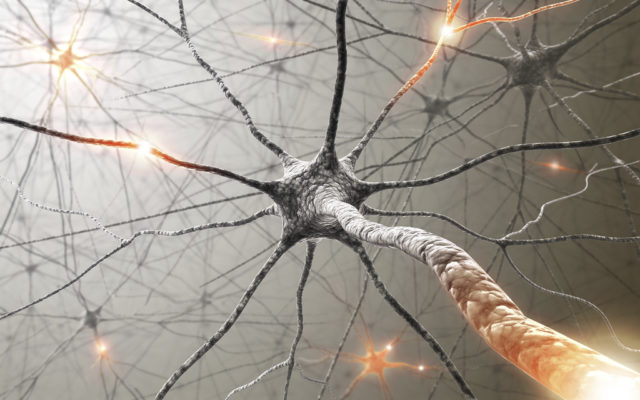
It comes down to genes – period.
To understand phases of circadian rhythms, we must also consider the genetic factors that control our habitual patterns. The first circadian rhythm gene to be identified (at first in fruit flies, but later found in many mammals) was the period gene. Expression of the period gene results in the production of the PER protein in mammalian SCN cells. PER protein accumulates throughout the night, resulting in an abundance of the protein that starts to inhibit its own production, switching off the mechanism that synthesises it to allow for the degradation of excess protein throughout the day. When night comes back around, the levels of PER are low, and so the cycle begins again, creating a self-sustaining feedback loop. Since this discovery, more genes and proteins have been identified that affect sleep/wake cycles – further details can be found in this review: 3. This mechanism acts as the metronome of the circadian rhythm, initiating the synthesis or inhibition of other hormones and molecules that affect body temperature, digestion and other functions.
Mellow Melatonin
The SCN clearly has a lot to answer for when it comes to our daily rhythms – but it is not the only gland responsible for maintaining our active cycle. The circadian rhythm produced by our SCN dictates the production of the hormone melatonin from our pituitary gland. Studies have shown that melatonin content in human plasma follows a trend similar to that of the PER protein – accumulating through the night and degrading again in the day. This is because melatonin is a photopigment – a molecule that is sensitive to light. Exposure to bright light in the middle of the night causes a sudden decline in melatonin as it reacts to the light and degrades. It also has sleep-inducing properties, so as levels of the hormone start to rise early in the night, this encourages our bodies to prepare for sleep. What’s more, there are melatonin receptors on the surface of some SCN cells, so an abundance of this molecule can also provide feedback to the master clock. This ongoing feedback helps the SCN to keep in sync with day and night.
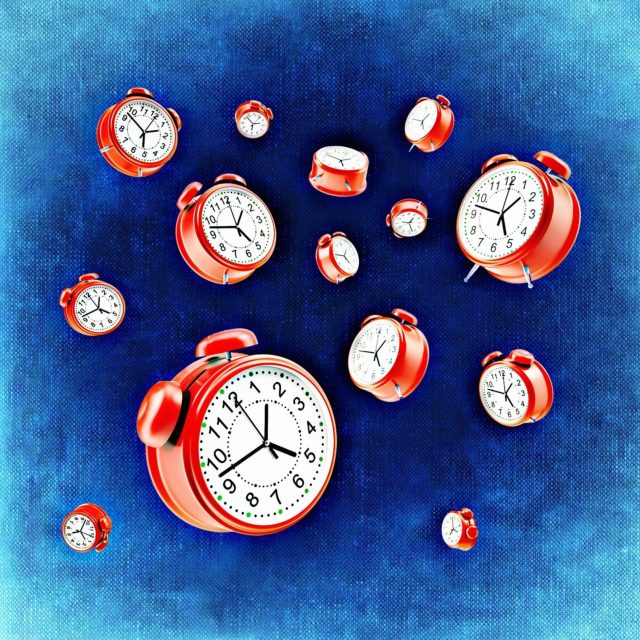
The Axis of Adrenal
Research into circadian rhythms of different species has shown that most mammalian systems are similar in structure and components. The environmental cues (known as zeitgebers) that encourage diurnal species to wake or rest simply have the opposite effect in nocturnal species. One factor that shows a clear definition between diurnal and nocturnal patterns is the activity of the hypothalamic-pituitary-adrenal (HPA) axis. This “axis” describes the complex interactions between the three glands, all secreting hormones that affect the body’s function throughout the sleep/wake cycle. In diurnal creatures, HPA axis activity peaks at the end of the dark period, whereas in nocturnal animals activity is highest as the light period changes from day to night. This suggests that the signals emitted from the HPA axis are a wake-up call for the animal to prepare for activity.
Evidently, the inner workings of our biological clocks are in fact a complex symphony, orchestrated by the different sections of our nervous and endocrine system to produce a flawless rhythm by which we live our lives. The similarity in mammals both nocturnal and diurnal is a testament to the robustness of this system, and also highlight the sensitivity of our natural routines to interference from artificial zeitgebers. So, if you are reading this on your phone before bed, stop right there! Turn off those lights, rest your eyes and let the melatonin flow…
This article was specialist edited by Holly Kerr and copy-edited by Gemma Donnelly.