Conquering the fast and the furious: Physics of atmospheric re-entry
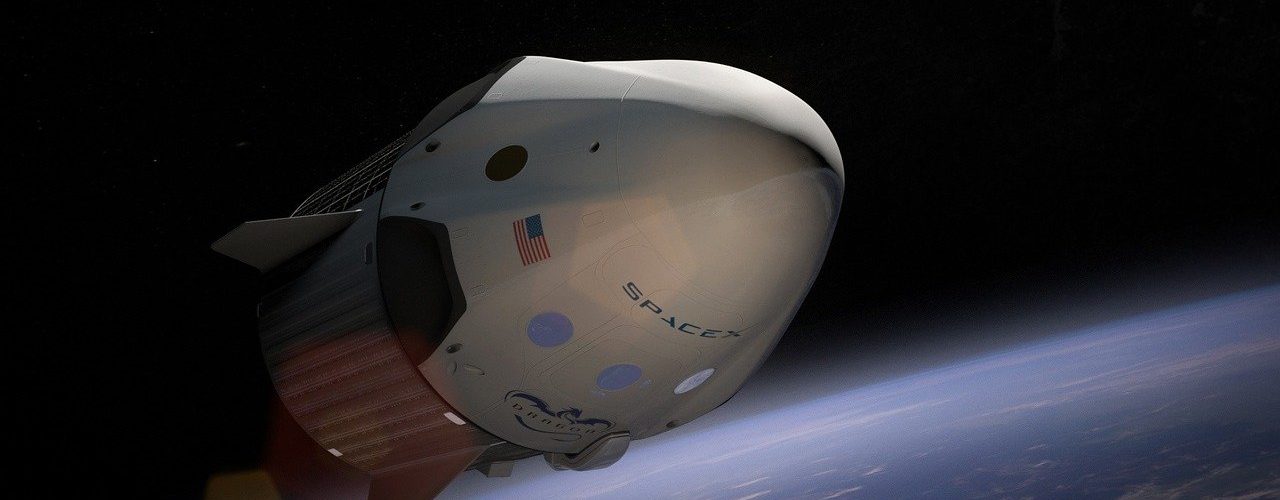
On May the 30th, SpaceX successfully managed to send a test space flight to the International Space Station (ISS). The crew of this collaborative mission, Demo-2, ushered in a new era of commercial space flight1. After spending over two months in space, on the 2nd of August, the two NASA astronauts who flew with this mission will return to Earth in the Crew Dragon capsule also developed by SpaceX. The re-entry of Crew Dragon into the Earth’s atmosphere will be equally challenging as sending them to the ISS. This is easily one of the most decisive periods which lasts only for a few minutes.
The physics of re-entry
Due to the extreme environment faced as a spacecraft descends towards Earth, the atmospheric re-entry is a crucial period of any space mission that involves the safe return of experimental samples and/or astronauts. The returning spacecraft needs to be in a specific Earth orbit before it can initiate its return journey. This return flight must occur in a narrow passage in space called the “re-entry corridor”. It is within this passage that the atmospheric drag on the spacecraft is large enough to let it fall to Earth instead of letting it veer off into space but not so large that the resistance it receives from the surrounding air destroys it. The space shuttle, as most of us still fondly remember, was a somewhat controllable spacecraft but most other re-entry vehicles do not have a lot of control on their trajectory as they start their return journey to Earth. Crew Dragon is one of those spacecraft that simply fall down to the Earth on a pre-calculated trajectory. Once the spacecraft has been carefully navigated to fall through the re-entry corridor, the thermodynamics comes into play.
The air, as we know, is composed of mainly two diatomic molecules: oxygen and nitrogen. Ordinarily, when air pressure is low, they remain in this diatomic state. However, under special circumstances like atmospheric re-entry, they behave differently. During re-entry, as the spacecraft falls under gravity and enters Earth’s atmosphere, the increasing density of the atmosphere combined with the tremendous speed of the spacecraft (of the order of 7-8,000 metres per second) causes a shock wave leading to an enormous rise in the pressure in front of it. At approximately 70 to 80 km above Earth, this pressure becomes so large that it causes the diatomic oxygen and (after some time) nitrogen to split causing further reactions. These reactions are extremely exothermic – meaning they release a lot of heat. This heat is so significant that we need special arrangements to protect what’s inside the spacecraft.
During this crucial period of high energy thermodynamic reactions, the spacecraft continues to fall and slow down gradually. This mechanism of re-entry is indeed by design, as it allows the spacecraft to slow down enough to land safely while converting its kinetic energy to thermal energy. This is the reason engineers pay special attention to deal with this heat and design a “thermal protection system”. There have been accidents in the past which have caused tremendous damage and loss of human life2.
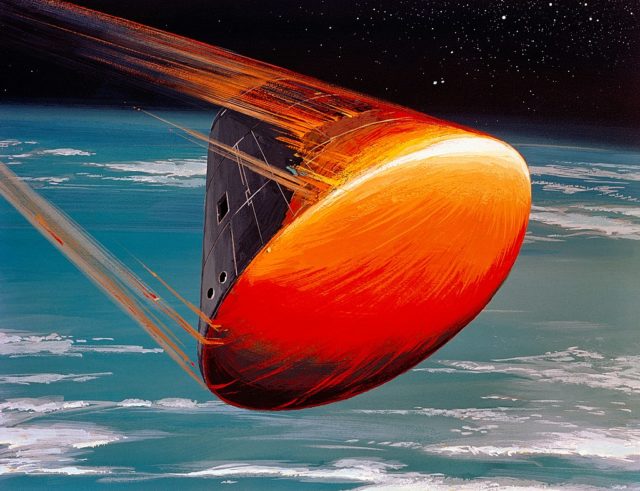
Image was created by North American Rockwell for NASA.
Another interesting effect of these physical phenomena is that because of the ionised state of air around the spacecraft during re-entry, the communication with the spacecraft does not work for these few minutes. This is termed as the “re-entry blackout”. It happens because the ionised air molecules interfere with the radio waves which we use to communicate with spacecraft. These moments of complete radio silence are therefore spent worrying and hoping that everything goes according to plan.
Brief history of re-entry thermal protection
The earliest techniques for re-entry, used during the Apollo era, were more rudimentary. The idea of adding more material to absorb the heat generated was one such example. This meant more weight had to be carried to space and it certainly was one of the expensive implementations. The Apollo heat shield was the beginning of a long race for a safer, cheaper, and more convenient thermal protection system. One of the next big programs by NASA, the space shuttle was a brilliant spacecraft primarily because it was reusable. For thermal protection, different types of special insulation tiles were used. These could be replaced if significant wear was observed. However, the technology used for the program proved to be exorbitant and the space shuttle flew for the last time in 2011. The much-hyped National Aerospace Plane was a single stage to orbit (SSTO) project in the United States and many new ideas were implemented in it. For the heat shield, it used the technology of heat pipes for cooling critical parts. Unfortunately, the ambitious project did not materialise due to multiple factors and was cancelled due to budget cuts in the early 90s.
Meanwhile, material scientists and space engineers came up with the idea of “ablation”. Ablation involves allowing the burning of layers of material used in the front of the spacecraft and in the process carrying the heat away with the burnt and disintegrated material. This way only the outer materials are exposed to the heat thus protecting the structure of the spacecraft. By the time the spacecraft lands, a portion of the front would have evaporated in air. This technique has been in use for a long time in combination with others. Essentially, this technique requires “refurbishing” the spacecraft to use again in the future and thus is certainly not reusable. One other more popular technique that’s still used is radiative cooling. In this technique, specific materials are used which allow the heat to reflect back into the atmosphere in the form of thermal radiation. So while the spacecraft accepts the heat, it is being released into the atmosphere simultaneously.
Over the years, as the technology developed, no standalone techniques have been used for the entire heat shield. Depending on the type of the re-entry spacecraft and location on the spacecraft’s body different insulating methods may be used in combination with these.
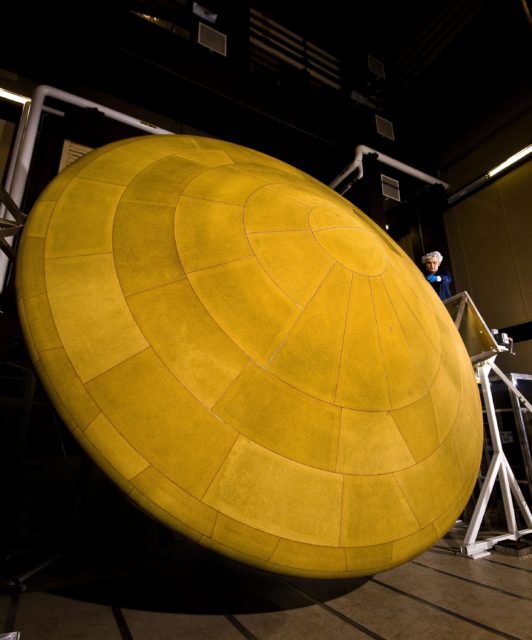
Transpiration cooling: A new way to reduce re-entry heating
Over the years, many alternative forms of thermal protection methods have been suggested and are being researched globally for a better thermal protection system. These range from the use of aerogels to the idea of magnetohydrodynamics. Transpiration cooling is one of these novel methods that came to the forefront of research in this decade, with many researchers experimenting and creating computer simulations to gain a better understanding. The idea of transpiration cooling itself is not new. The technique has been used earlier in less extreme applications like gas turbine cooling, however synthesis of some newer materials in recent times has allowed it to be reviewed in the context of atmospheric re-entry.
Transpiration cooling is essentially the use of a coolant to flow over the heated surface. This is achieved by passing the coolant through a layer of porous coating. The porosity allows the coolant to spread evenly and create a cold fluid layer in the front. This creates a barrier between the structure of the spacecraft and the external hot flow. The coolant heats up to shield the surface from incoming heat. With the development of newer porous materials which can withstand higher temperatures and pressures in the previous decade or so, researchers are now working on making the concept of transpiration cooling a reality. The idea is being examined from various aspects for a better understanding of the physics involved and its practicality. However, from the preliminary results, the concept looks very promising and could prove to be useful in future space missions. Recently, SpaceX’s Elon Musk announced3 that transpiration cooling will be utilised for critical areas of the Starship spacecraft. In the coming years, we will see how it turns out!
This article was specialist edited by Miriam Payne and copy-edited by Dzachary Zainudden
Very Interesting info. As per the paper the technic seems to be sound. Time will give the results.
Well done Deep
“7-8,000 kilometres per second” is incorrect by a factor of 1000.
Thanks for the spot – supposed to be metres and not kilometres! Correcting now.
Very informative. It’s always interesting to read something about human space relationship.