Helping Fractures Heal
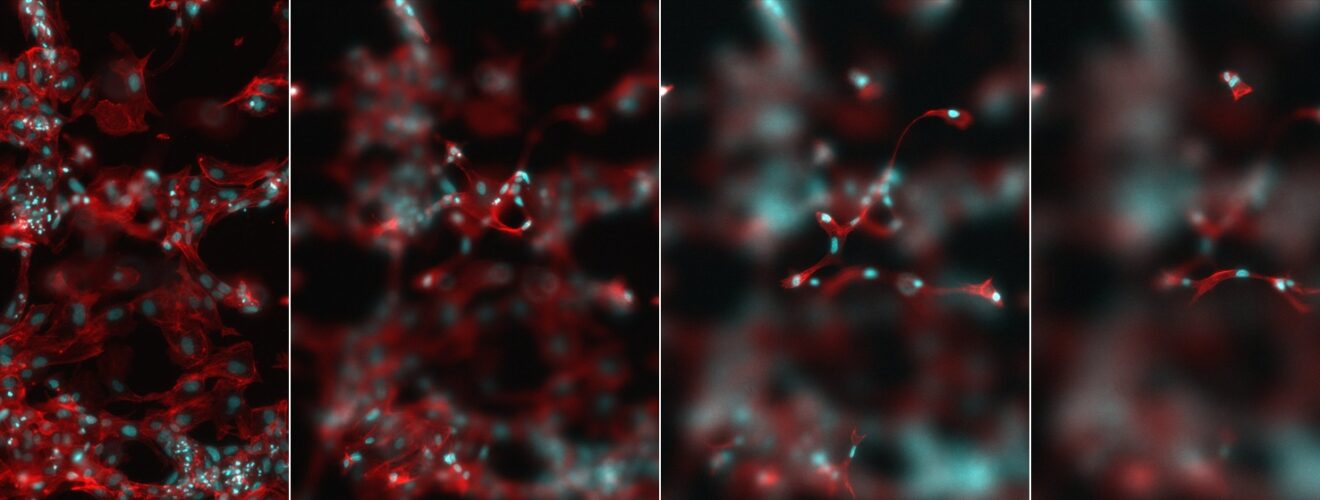
Imagine, it’s early June 1667 and you’re a Dutch soldier at the mouth of the river Thames. You‘re from a newly created marine unit that is one of the first European military units specialising in amphibious operations and your side is winning. Unfortunately, you’ve just been shot in the head.
Usually a wound like this would be fatal. Fortunately for you the lead ball from this musket only skimmed your head. It took out some bone from your skull, but didn’t touch your brain, and you are now in the hands of some of the most advanced doctors of your time. The next few months are tortuous, filled with wound cleaning and recovery time, but your skull doesn’t fully heal. Your doctor, Jacob van Meekeren, has a revolutionary idea. He wants to use a piece of bone from a dog to close your wound. Nobody has ever done a procedure like this before. It is the first recorded bone graft in history.
Bone is one of the few human tissues that can regenerate by itself. All bone mineral has a scaffold-like structure that bone cells enjoy attaching themselves to and growing on. So the dog bone graft worked, and the soldier recovered successfully. The graft worked so well that van Meekeren was unable to remove the piece of dog´s bone when the soldier asked him to the dog’s bone had fused to the soldier’s skull. The soldier lost his job. Following his miraculous recovery, he had been excommunicated by local religious leaders for being ‘part-dog’ and therefore he wanted the bone removed. Ultimately, however, he decided that he preferred not being dead to being ‘part dog’.
Today, bone grafts are still used for fractures that don’t heal properly. The surgeon will usually cut a piece of bone from somewhere in the body that isn’t regarded as ‘critical’ and use it to bridge the gap in a fracture. If this option is not available, the surgeon can use synthetic materials to replace the bone. Our understanding of how we can help bone fractures heal has increased so much during recent years that synthetic grafts are sometimes as good, or even better than natural bone. These advances are being used in the clinical setting to improve orthopedic implants.
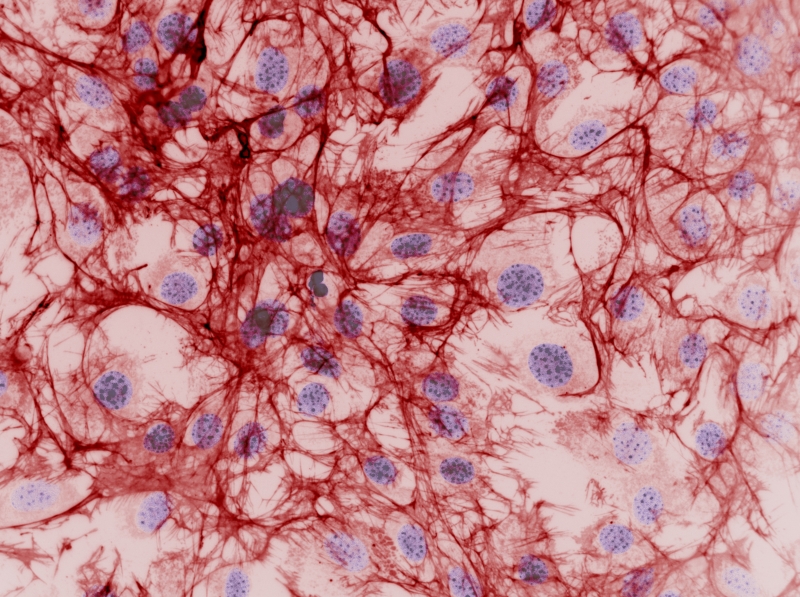
Fibronectin matrix surrounding cells in an in vitro culture. Image credit: Cristina Gonzalez and Marco Cantini.
Research into natural regeneration and healing started to influence clinical applications about two decades ago. This has helped patients of trauma, spine fixation, craniomaxillofacial and joint replacements, as well as in other tissue engineering fields. Today, orthopaedic surgeons are able to use artificial matrix materials such as calcium phosphate (that can form a structure that closely resembles the mineral part of bone) together with growth factors (proteins that are able to recruit stem cell to the injury site) to direct the healing process. Discovered more than fifty years ago in a myriad of natural biological processes, growth factors are mass produced today through genetic engineering. Their use is, however, rather controversial as the high concentrations used in current applications have potentially undesirable side-effects.
To overcome these hurdles, current research is looking into ways of using growth factors more safely, or using synthetic materials with bioactive properties to guide stem cell fate and bone regeneration. This is the goal of several research labs at the University of Glasgow.
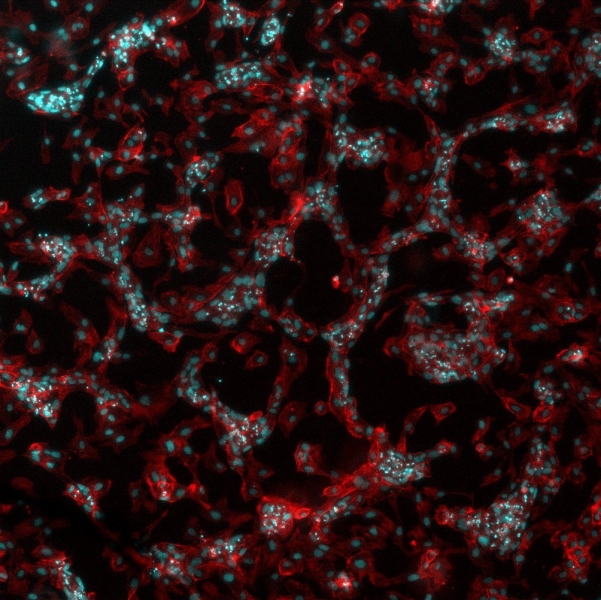
HUVEC cells stimulated with VEGF on a polymer surface. Image credit: Vladimira Moulisova.
Professor Liz Tanner recently received an OBE for her thirty year career and work in bone augmentation and replacement research, such as developing composites of polymers and bioactive ceramic materials to be used as bone substitutes. She authored a review on the properties of phosphate glass fibres and composites recently published in the journal ‘Biomedical Materials’. These are a family of materials of particular interest because, not only are they able to induce and conduct bone growth, they can degrade in vivo at a controllable rate; making room for real bone to grow in its place.
A breakthrough series of papers published between 2007 and 2014 in the journal ‘Nature Materials’, by University of Glasgow researchers led by Professors Matthew Dalby and Nikolaj Gadegaard, describe how surface topography at the micro and nano scale has an effect on the behaviour of stem cells. This work has explained cellular mechanisms by which specific patterns on the surface topography guide the conversion of human mesenchymal stem cells (hMSCs) to osteoblasts (cells that form bone), successfully driving differentiation towards the production of bone in vitro. This could be used, for instance, in advanced implants to improve the integration and performance of hip, knee, and dental implants, among others.
Cells interact with the surface of the materials they grow on through a variety of proteins in their membranes, surrounding them in what it is called the extracellular matrix (ECM). Growth factors can be found in the ECM, carrying important messages for the cells that encounter them. Researchers are aiming to understand and engineer these cell-material interfaces by controlling the way ECM proteins and growth factors are presented to the cells, to induce the start of the complex, natural, healing microenvironment.
University of Glasgow Professor Manuel Salmeron-Sanchez has received European ERC and UK Regenerative Medicine Platform awards to study how synthetic materials can trigger particular changes of ECM proteins in a biomimetic way that improves the effect of these proteins and growth factors on stem cell differentiation. Together with Prof Dalby’s team, they are working to translate this research into clinical orthopedic applications. This means a reduced dose of growth factors would maintain the same effect in bone healing – avoiding the side-effects of using high concentrations.
Prof. Dalby’s team is also working on a different approach, “nanokicking” stem cells using high frequency vibrations to stimulate bone formation. Cells that are forming new bone tissue receive signals from their neighboring cells in the form of vibration between their membranes. The nanokicking bioreactor ‘kicks’ the stem cells in the lab around 5-30 nanometres in distance 1,000 times a second, encouraging bone formation.
These, and many other research labs, are working on novel cell-based therapies, using stem cells harvested from the patient or from donors. Cell therapies are still not extensively approved for clinical uses, but have delivered encouraging results at the research stage, and could potentially revolutionize treatment for orthopedic conditions and many others.
This article was specialist edited by Gwenllian Tawy and copy edited by Nicole Nayar.