Follow the Arrows
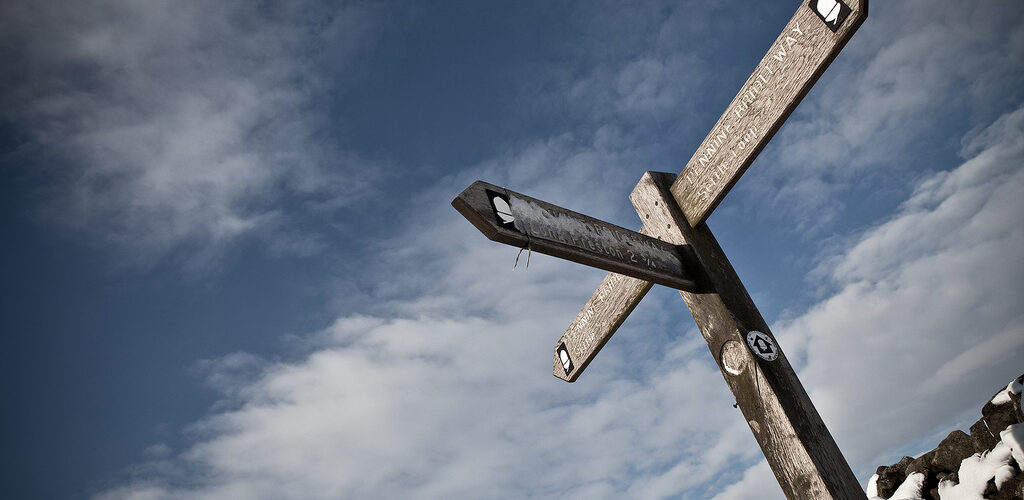
‘How did we get here?’
An important question both philosophically and scientifically, depending on your angle. If, like me, you are more inclined to postulate science-related matters, perhaps you mean how did life begin on this Goldilocks planet, where the environment is ‘just right’ for life, or even how is it that the known universe came to be formed? If you’re even more like me and spend a lot of time thinking about this question in a chemical sense, maybe you’re looking at the mechanisms in your organic chemistry textbook and thinking:
‘I have no idea how this changes into that. Seriously, how did we get here?!’
But then again, maybe you’re not.
Whilst I am certainly no expert on how it is that we all got here (in whatever sense), I am a little more clued up on chemical transformations and how they can shuttle the structure of your molecule from point A to point B, C, D or even X, depending on the number of chemical steps required.
Theory first
The disconnection approach, a theoretical tool used by organic chemists to break up large molecules into smaller starting materials, can help with designing potential routes to the target molecule. However, this is only helpful if the chemist understands how to utilise this knowledge, and is able to rationalise how to get from the starting materials to the end goal by choosing appropriate reagents and reaction conditions; this is where reaction mechanisms come in.
Ah, push it (oh baby, baby)
All chemistry students are familiar with organic mechanisms, whether they want to be or not. They are a fundamental part of describing the chemistry behind the reactions, and serve as a great teaching tool. Many an hour is spent drawing out starting materials and other reagents on a page and using ‘curly arrows’ to push electrons around in order to obtain the final target structure. Lots of scribbles, crossing out, crying and re-drawing before getting it right tend to accompany any venture into university level organic chemistry. The arrow pushing is used to detail the movements of the electrons and help visualise which bonds are breaking and where new bonds shall be formed over the course of a reaction. A one-headed arrow is used to indicate the movement of one electron as in a radical reaction, and a two-headed arrow is used when considering the movement of two electrons (the maximum number of electrons in a single chemical bond). When using line or skeletal formula as in Figure 1 below, two electrons in a bond between two atoms are represented as a straight line between the atoms.
A good but simple example to consider is that of the Diels-Alder reaction, which sees a diene (a molecule containing two double bonds) and a dienophile (which contains at least one double bond) react together to form a 6-membered ring. The arrow pushing for this reaction is very neat, and, as there are typically only two participants in the reaction, the mechanism can be drawn out very simply like so:
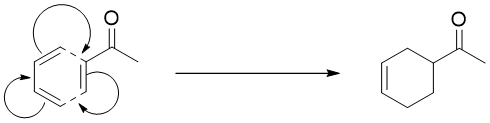
A typical ‘curly arrows’ mechanism for the example Diels-Alder reaction. Left to right: An example of a diene and a dienophile, followed by the resulting cyclic product on the right hand side. The dashed lines represent the new bonds that are forming. Image by Debbie Nicol
Of course, mechanisms can be drawn without the arrows, but using them offers the advantage of being able to keep track of what electrons are being transferred where and which bonds are being broken or formed. This is particularly useful if there is a lot going on simultaneously. (Take it from a chemistry undergraduate – curly arrows are your best friend during exams.)
So when will you actually use all the stuff that you learned at uni?
Over the years, there has been much speculation as to whether there is any sort of basis for curly arrow-led mechanisms. The mechanisms taught to chemistry students may have little or no physical or chemical basis in terms of how a reaction actually progresses – it is difficult to work out the mechanism for reactions that lack [detectable] intermediates, particularly if several compounds are involved. Which species reacts first with what? What happens then? Does this happen before or after that, and at what speeds do the reactions progress at? Mechanisms tend to be accepted if they support known chemistry and are consistent with available experimental evidence, but there have been no reports of a discernible link between curly arrows and quantum chemistry – until now.
So, as it happens, it seems that the answer to the most important question – is this all worth learning? – is actually yes.
Earlier this year, Knizia and Klein published a paper entitled Electron Flow in Reaction Mechanisms – Revealed from First Principles, in which they show that there is consistency between computationally calculated mechanisms and their corresponding curly arrow mechanisms 1. In their paper, it was noted that only three other articles had been found that had previously attempted to tackle the physical basis of curly arrows, one of which concluded that there was no basis for such mechanisms (Gineitytė, Lit. J. Phys., 2011) 2. However, Knizia and Klein’s work took a more in-depth approach to the computational chemistry, improving the computation to include information on which steps during the reaction were more energetically favourable, and so more likely to happen. Previous work by Klein in the same area suggests that the new computational method is applicable even to complex systems. As such, the way we determine reaction mechanisms could rely ever more heavily on computational calculations as opposed to just chemical common sense.
So, how did we get here? Where did all this chemistry come from? And why have I drawn so many hexagons trying to figure it out? Perhaps the answer lies in computation – it would seem that chemistry is no exception to the steady advance of STEM (science, technology, engineering and mathematics) subjects through the use of computer modelling. So for now, if you are ever confused, I’d recommend that you follow the arrows – they should keep you right.
This article was specialist edited by Kirsty McLean and copy edited by Rebecca Baird and Emilie Steinmark.