Frozen Solid
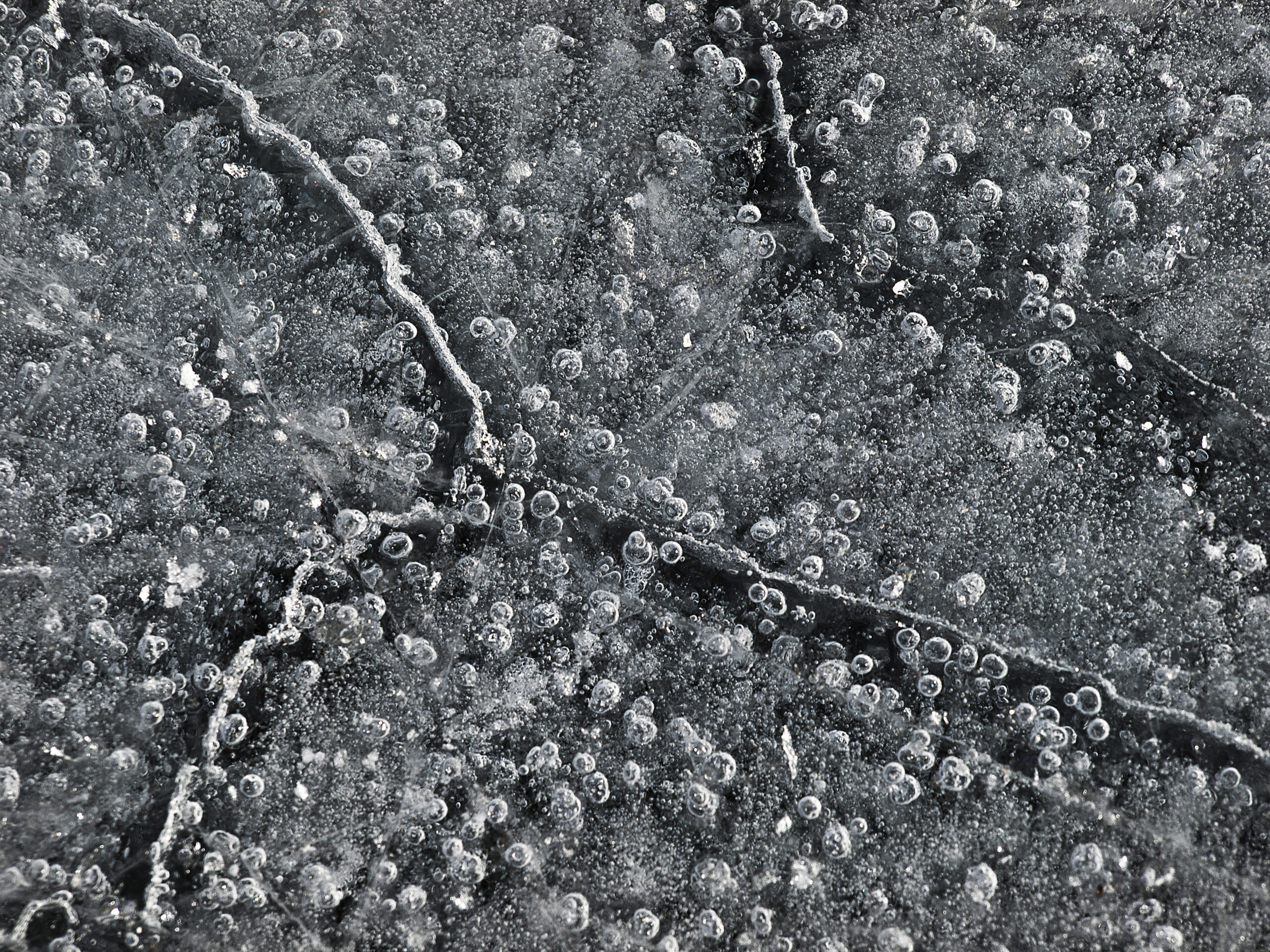
The Nobel Prize recognises great achievements in science, culture and academics and celebrates tremendous advances in society, with 2017’s award in chemistry being no exception. The prize was presented to Jacques Dubochet, Joachim Frank and Richard Henderson for developing a technique that has led to vast improvements in the imaging of large biological molecules. This technique is called Cryo-Electron Microscopy (Cryo-EM) and it is an extremely powerful investigative tool, allowing scientists to see the biological structures of macromolecules, such as proteins, in high resolution. The images produced from this innovative approach has propelled the development of drug discovery by allowing us to predict how drugs will interact with their target molecules and has contributed to the production of potent medications, keeping us at the very cutting-edge of science. However, before we look at exactly how it works we need to understand what cryogenics is.
When the word temperature is used, it generally refers to how hot or cold something is. However, when scientists use the word temperature we are referring to the motion of particles. The temperature of a system, whether that is a liquid, solid or a gas is a measure of the average amount of kinetic energy that the particles in that system have. Furthermore, a greater temperature corresponds with a greater average kinetic energy.
The Kelvin (K) scale defines the absolute temperature scale where 0K is the point at which particles have the least possible motion. As such, they can get no colder and this holds regardless of the properties of the substance we are measuring. Scientists generally accept that when temperatures of 123.15K (-150ºC) are recorded, materials such as liquid nitrogen or helium exhibit cryogenic behaviour.
In comparison, the lowest dependable ground measurements recorded were at the Vostok research station in Antarctica, where temperatures reached -89.2ºC1, so we have would have to have an almost two-fold reduction of that result before we’d be within cryogenic range!2
It was Jacques Dubochet who first pioneered the use of these cryogenic materials to resolve the issue of sample degradation and allow for more sophisticated images of molecules such as proteins and nucleic acids to be obtained. He was frustrated by how much biomolecules would lose their structure and degrade when placed in the vacuum of an electron microscope and spent much of the early 1980s researching this problem with his team at the University of Lausanne. Commonly, transition electron microscopes (TEMs) are used to determine the structures of molecules. Instead of using light, with these microscopes, electrons provide an insight into the arrangement of the materials.
Dubochet recognised that, problematically, the intensity of the beam as well as the high-vacuum setting led to the destruction of certain samples that seemed to be unsuited to this technique. As such, a more innovative method was needed to avoid these complications and to produce clear molecular images.
Five years earlier, whilst based at the Laboratory of Molecular Biology in Cambridge, Richard Henderson was working to refine the technique of producing images using TEMs. He was also frustrated by the limited imaging capabilities that alternative techniques such as x-ray diffraction or nuclear magnetic resonance (NMR) offered. Both methods had the benefits of generating high-resolution models of the structures of biomolecules. However, NMR works best when small, soluble proteins are used, which doesn’t include many found in cell membranes, and x-ray diffraction requires crystallisation of the sample, a process which many proteins cannot undergo. Henderson quickly focused his attention on developing a 3D structure of a membrane protein called bacteriorhodopsin, refining his technique until clearer and clearer images could be cultivated.
The problem still facing scientists was how to achieve the resolutions needed to produce highly detailed images of cells, the kind that would rival x-ray diffraction’s success with crystallised samples. Using the TEM’s concentrated beam was found to evaporate the water contained within the cell and thus destroy its structural integrity, so even trying to view the cell would cause extensive damage. Furthermore, standard freezing techniques caused ordered ice-crystals to form, diffracting the beam of the microscope and producing a highly distorted image. An innovation was needed and Cryo-EM provided just that. Dubochet’s ingenuity came from flash-freezing the cell through a process called vitrification whereby the cell becomes glass-like instead of forming a crystalline structure. Using vitrification and a less concentrated beam, he pioneered a process whereby the sample is placed on a metal screen allowing a very thin film of material to be obtained. This film is then fired through ethane and cooled to -190ºC, allowing the water to be vitrified. All that remained was to pass the sample into the TEM and cool it with liquid nitrogen. By using a detector it was then possible to view the structural image of the biomolecule.
The final piece of the puzzle came from Joachim Frank’s cutting edge algorithms and imaging analysis. His brilliance was to take thousands of pictures of proteins which had been hit with a beam from a TEM from a variety of angles and layer them, creating an incredibly detailed 2D picture. Frank’s development of computational techniques then allowed a 3D image of his 2D projections to be built, culminating in a high-resolution image of the structure of a biomolecule3.
The individual work of these visionaries has allowed for great advances in the fields of structural biology and biotechnology as well as the pharmaceutical and healthcare industries. Cryo-EM has revolutionised the storage of blood used in emergency medicine and the transportation of food over long distances to be used in humanitarian aid efforts. Recently, Nature published a study 4 detailing the imaging breakthroughs made in the search for a treatment for the neurodegenerative disease Alzheimer’s. This is something that would have not been possible without this pioneering technique and is why the development of Cryo-EM has been a pivotal step forward, one truly worthy of a Nobel Prize.
As the search for new drugs to combat increasing bacterial resistance continues, techniques like Cryo-EM are becoming invaluable. Scientists from the University of Groningen conducted research in 2017, investigating structural changes and identifying the mechanism of a particular ribosome in the bacterium Lactococcus lactis 5 that increases resistance to antibiotics. This vital report highlights the challenges involved in antibiotic resistance, and the reliance on Cryo-EM as a vital analytical tool in the development of drugs to target this intensifying issue.
Professor Linse of Lund University in Sweden and chair of the committee awarding the prize for chemistry was recently quoted as saying “Soon there are no more secrets,”6 and with the technique advancing so many scientific endeavours it is hard to disagree. The outstanding work of Richard Henderson, Joachim Frank and Jacques Dubochet has made it possible to study the structures of molecules intricately and it is clear that cryogenic materials will be powering our future for many generations to come, allowing us to gain hugely exciting insights into science previously undiscovered.
This article was specialist edited by Derek Connor and copy edited by Kirsten Woollcott.
References
- https://www.bas.ac.uk/data/our-data/publication/record-low-surface-air-temperature-at-vostok-station-antarctica/
- A very useful video helping us to understand these temperature scales can be found here. https://www.khanacademy.org/science/in-in-class11th-physics/in-in-thermal-properties-of-matter/in-in-temp-kinetic-theory-ideal-gas-law/v/absolute-temperature-and-the-kelvin-scale
- https://www.chemistryworld.com/news/explainer-what-is-cryo-electron-microscopy/3008091.article
- https://www.nature.com/articles/nature23002
- https://www.nature.com/articles/s41467-017-00718-x
- https://www.nytimes.com/2017/10/04/science/nobel-prize-chemistry.html