Antibiotic Resistance: The arms race between host and pathogen
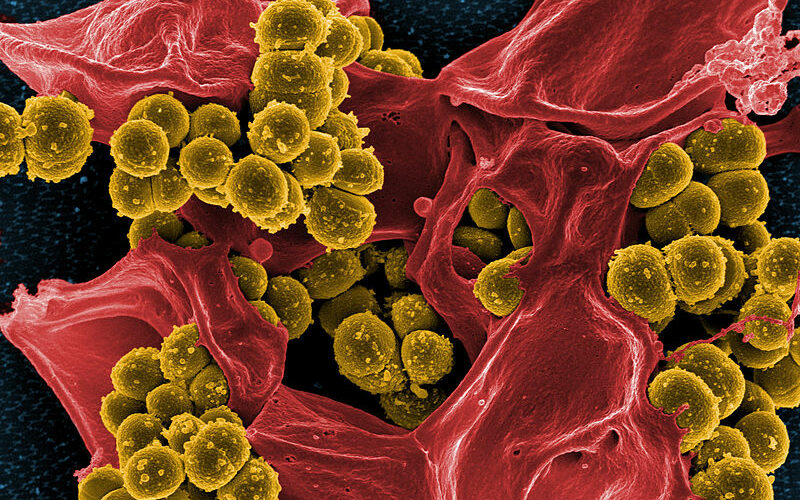
Antibiotics are without doubt one of the major medical discoveries of the 20th century and have significantly contributed to the control of infectious diseases around the world. Alexander Fleming’s discovery of penicillin, together with the visions and contributions of scientists like Paul Ehrlich, heralded the start of the “antibiotic era” and smoothed the path towards the development of essential medical procedures. With the ability of surgeons to control wound infections following major and minor surgeries and the treatment of many other bacterial diseases, came a significant decrease in the mortality and morbidity associated with these infections, leading to modern life as we know it today 1.
But what exactly are antibiotics and how do they work? In general, the term ‘antibiotics’ can be used interchangeably with ‘antimicrobials’, and encompasses a large group of molecules that can disturb the growth of their target bacteria by interfering with important cellular processes. For example, bacterial cells have strong walls, which serve as a barrier between the cells and anything in the environment that can harm them. These walls are continually being rebuilt and some antibiotics have the ability to impede cell wall synthesis, preventing bacterial growth. Nowadays, resistance to these lifesavers is spreading all over the world, leading to an increase in morbidity and mortality due to bacterial diseases.
But how is it possible that certain bacteria can become insensitive to antibiotics? Bacteria, like mammals, are living organisms and thus have to be able to carry out basic biological functions. One important feature that mammalian and bacterial cells have in common is that their DNA code determines all of their cellular functions, and much like our own genes, bacterial genes can mutate. Bacteria have a special feature that makes them extremely successful in this respect – their small genome is dynamic and prone to changes, which allow them to adapt to external selective pressures. In principle, there are two ways to acquire antibiotic resistance: either through mutations in the bacterial DNA or through neighbouring donor bacteria who can pass on part of their DNA containing antibiotic resistance genes to a recipient bacterium 2. It is also possible to gain resistance to many of these antimicrobial weapons. The increasing occurrence of these multiple drug-resistant clones is a major problem, as it restricts the possible treatments to only a few, often more toxic antibiotics. But these last-resort antibiotics are also starting to fail, leaving doctors increasingly helpless against the intelligence and fast adaptability of these bacteria. Resistant strains such as Staphylococcus aureus and carbapenem-resistant Klebsiella pneumoniae are increasingly common in Europe, and are considered a major threat not only to society but also to animal health, with increasing economic costs 3.
In addition to a growing awareness of resistance and restricting the usage of antibiotics, the fight against these ‘superbugs’ has to be strengthened with the discovery of antibiotics with new mechanisms of action, and the improvement of currently available antibiotics. In January 2015, a new antibiotic called teixobactin was introduced to the scientific world by a research team led by Kim Lewis, from the Northeastern University in Boston. Using a new technology to grow bacteria that were previously uncultivable, this molecule was isolated from the soil bacterium Eleftheria terrae. It caught the attention of the press because no development of resistance was observed during their experiments, however this doesn’t mean that resistance won’t arise in the future 4.
As potent as these novel antibiotics may be, they may face the same resistance issues in the long run, so there is still an urgent need to find alternative treatments. Therefore, scientists have been trying to exploit specific behaviours and features of pathogenic bacteria such as biofilms and other bacterial virulence strategies.
Bacteria like Staphylococcus aureus use clever mechanisms to investigate their surroundings and communicate with other bacteria, which is often the basis for the successful establishment of infection. This happens through the secretion of small molecules by one bacterium, which can then be detected by other bacteria. This makes it possible for them to guess the number of bacteria in their surrounding area and can ultimately result in multicellular, coordinated actions – a process called “Quorum sensing”. Quorum sensing is needed for biofilm formation. Biofilms represent multiple layers of adherent bacteria, which act together to form a community of specialised cells and are often hard to treat with common drugs. They are a particular threat in hospital-acquired infections as they can also be established on non-biological surfaces, for example catheters or surgical instruments. This highlights a new direction that antimicrobial strategies could take: disturbing these organised communities, which could lead to an increased susceptibility to antimicrobial substances and facilitate the eradication of an infection 5.
However, different bacteria can have different collections of weapons or abilities to cause disease. Another example is the needle-like secretion system used by E. coli to inject bacteria-specific effector proteins directly into a human cell, leading to altered cell behaviour. This can result in increased colonisation and adherence to the tissue, for example the intestine, leading to disease symptoms. Interfering with the activity of these bacterial weapons might show great potential, as it could disarm the bacteria and reduce their ability to colonise their host 6.
Viruses are also a great threat to health; humans as well as bacteria can be targeted and harmed by these minimalistic and efficient pathogens, which led to the idea of using them as an antimicrobial therapy. Some viruses, called bacteriophages, nest inside bacteria and hijack their resources which, while detrimental to bacteria, could potentially be beneficial to us. As a matter of fact, the idea of using bacteriophages to target specific bacteria dates back to the first half of the 20th century, but was widely neglected due to the discovery of antibiotics. Their key advantage over broad-spectrum antibiotics is that they can exclusively target disease-causing bacteria. Although interesting for many companies, the use of phage therapy still faces problems that are yet to be overcome 7.
Another possibility is the use of antimicrobial peptides. These small protein molecules are part of the immune system of mammals and bacteria, and are mostly known for their disruption of the bacterial cell membrane, which ultimately leads to bacterial death. Although mammalian cells are protected from these peptides by the slight difference between their own cell membranes and the ones surrounding bacteria, toxicity was reported in some cases when they were used in high concentrations. Bacteria-derived antimicrobial peptides like Nisin are also called bacteriocins, and are already used in food conservation. Additionally, researchers at the University of Michigan recently proposed that they may show anti-cancer activity in addition to activity against resistant ‘superbugs’. Other pathogens such as parasites, fungi and viruses are also possible targets of antimicrobial peptides, showing the broad possible usage of these molecules 8.
Right now, these approaches together with coordinated and effective strategies are needed to ensure that we don’t find ourselves in a world where even minor infections can be fatal, and doctors aren’t able to undertake surgery or treatment without risking patients’ lives. Nevertheless, current approaches and discoveries in this field show that research is on its way to address the issue on different fronts, and current social and political actions show an increasing awareness of this complex challenge.
This article was specialist edited by Teodora Aldea and copy edited by Nia Linkov.
References
- Aminov, R. I. (2010). A brief history of the antibiotic era: lessons learned and challenges for the future. Frontiers in microbiology, 1, 134.
- http://text bookofbacteriology.net/res antimicrobial_3.html
- http://ecdc.europa.eu/en/healthtopics/antimicrobial_re sistance/pages/index.aspx
- http://www.nature.com/news/promising-antibiotic-discovered-in-microbial-dark-matter-1.16675 Original publication: Ling et al. (2015). A new antibiotic kills pathogens without detectable resistance. Nature, 517(7535), 455-459.
- Marshall, J. (2013). Quorum sensing. Proceedings of the National Academy of Sciences of the United States of America, 110(8), 2690. http://doi.org/10.1073/pnas.1301432110
- Gu, L., Zhou, S., Zhu, L., Liang, C., & Chen, X. (2015). Small-Molecule Inhibitors of the Type III Secretion System. Molecules, 20(9), 17659-17674.
- Fernebro, J. (2011). Fighting bacterial infections—future treatment options. Drug Resistance Updates, 14(2), 125-139.
- Shin, J. M., Gwak, J. W., Kamarajan, P., Fenno, J. C., Rickard, A. H., & Kapila, Y. L. (2015). Biomedical Applications of Nisin. Journal of applied microbiology.