Can Neutrino Oscillation Explain the Universe?
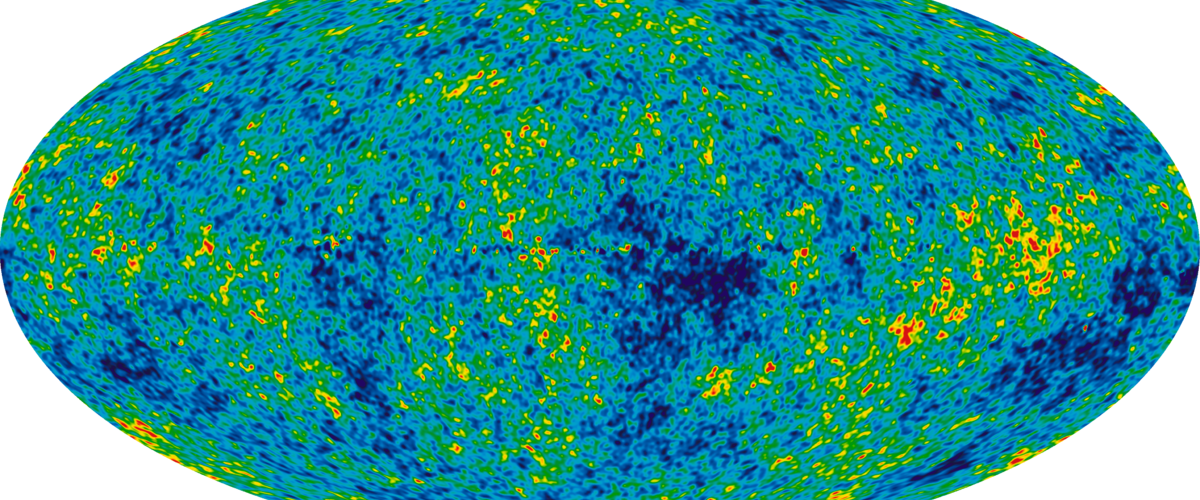
“Where did we come from and why are we here?” The search to answer these questions has guided our scientific endeavours for centuries. Many theories were postulated, and the answer evolved accordingly until the big bang theory was developed. This theory states that everything in the universe came from an initial burst of energy, and would explain a lot of the phenomena we see in the universe. One of the main concerns is the fact that this burst of energy should have created both matter and antimatter in equal amounts. We see this in the particle collisions at CERN where we create matter and antimatter from energy. It should be noted that this goes both ways – when matter and antimatter meet they create pure energy. Since there is not the same amount of antimatter as matter in our solar system, the question becomes: “where is the antimatter?”. This issue is known as baryogenesis, or leptogenesis, depending on what particle is in focus. Thankfully there are theoretical models to explain how baryogenesis can be solved through leptogenesis, see for instance 1, meaning that it may be enough to study leptogenesis alone.
Astronomers have explored the universe around us and found about one antimatter particle for every billion matter particles. 2. One question to ask from this is “have they looked in the right places?”. They have not been able to investigate the whole universe, however, if there was antimatter in the universe there should be signs in the background radiation and a light ‘fingerprint’ where the matter and antimatter meet and annihilate each other. Since there is no sign of annihilation, the main focus becomes looking for an interaction that is different for matter and antimatter. This would provide a means for matter to survive while antimatter is destroyed. One of these methods is neutrino oscillation.
Neutrinos are tiny particles that only interact through the weak nuclear force, and because of their miniscule chance of interacting, millions of them pass through us every day without us noticing. There are three d
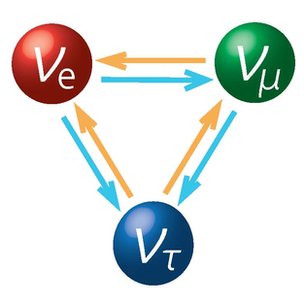
Credit: BBC.
Neutrinos can oscillate between their different flavours.
ifferent types, known as flavours: muon, tau and electron, relating to what interactions they partake in. Only a neutrino and an antineutrino of the same flavour will annihilate. Neutrinos have been of interest since their discovery in the early 20th century when they were assumed to be massless. Through the discovery of their different flavours and their oscillations we learned that they must have mass. Oscillations mean that they have the ability to change between their different flavours over time. This discovery was awarded the nobel prize in 2015 3 and experiments in 2002 showed the possibility of neutrino oscillation 4. This means that one can interpret a neutrino as having all of the flavours at once, but depending on when you measure it you will get only one of the flavours. This is similar to Schrödinger’s cat, where only one measurement is given but the state is in two different levels until measured, either alive or dead.
If there is a difference between how neutrinos and antineutrinos oscillate, then that would lead to the possibility of the neutrino and antineutrino having different flavours when interacting and therefore not annihilating. This would lead to free antineutrinos which can be used in inverse-beta-decay, where a proton, electron and antineutrino interact to create a neutron. So finally these surviving neutrinos will result in a neutron and a free neutrino, both of which are normal matter particles. If the oscillation difference is large enough, then this would explain why more matter than antimatter is seen in the universe. In general the difference between interactions of antiparticles and particles is known as charge and parity violation or CP-violation. Charge violation comes from the definition that anti-particles have a different charge, and parity since we see antiparticles as normal particles which are moving backwards.
Neutrinos and antineutrinos are being researched to find differences in their oscillations, however, as of yet there are no conclusive results to show that there is a difference. This is mostly due to the fact that there are currently no experimental setups sensitive enough, however there are plans to build facilities with enough sensitivity (see for instance the information about a European neutrino factory 5 as well as the CERN neutrino platform, where my current experiment is listed: babyMIND 6.) With a multitude of experiments currently underway, soon the question of whether neutrinos can solve the matter-antimatter imbalance will be answered and new physics can be explored.
To summarise, neutrinos are particles with an extremely low mass which began as a chargeless, massless, mathematical construct that helped to explain beta-decay. Interest continued through the start of the 20th century where their different flavours were discovered and experiments were set up to investigate lower levels of a specific flavour than expected. These experiments led to the theory of neutrino oscillations, which requires at least one neutrino to have mass, to now trying to understand and measure these oscillations and masses. If oscillations are measured as being different between neutrinos and antineutrinos (CP-violation), it will solve one of the currently unsolved problems with the big bang theory.
In recent years, a long list of previously unsolved problems in physics have been solved. The discovery of the Higgs boson and gravitational waves were enormous breakthroughs in physics and astronomy, shoring up theories and laws upon which some of our current technology is based. When we do finally solve the matter-antimatter imbalance, it will support the big bang theory, and we will be one step closer to understanding the tapestry of the universe. All in all, it is an amazing time to be involved in physics.
This article was specialist edited by Paul Walker and copy edited by Nina Divorty.
References
- https://arxiv.org/abs/hep-ph/0608347
- A good article describing the current search and prospects can be found athttps://arxiv.org/abs/1306.4171
- http://www.nobelprize.org/nobel_prizes/physics/laureates/2015/
- http://www.nobelprize.org/nobel_prizes/physics/laureates/2002/
- http://www.ids-nf.org/wiki/FrontPage
- http://cenf.web.cern.ch