Green Chemistry: An Elegant Solution to an Avoidable Problem
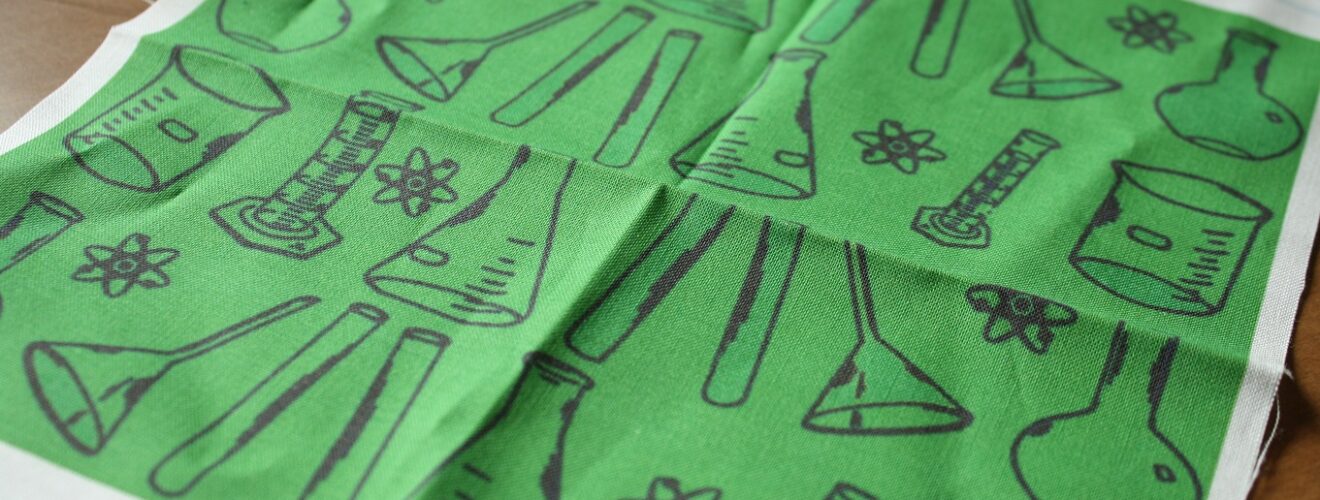
Let’s save the environment. Pick up that litter; utilise public transport and help reduce the emissions of greenhouse gases; use energy-saving light bulbs; recycle!
The above message, whilst an open invitation to everyone (and a very welcome one too), is particularly aimed at those interested or involved in synthetic organic chemistry, whether in an academic or industrial role. For many years, the chemical industry in particular hasn’t exactly had the best relationship with the environment. There have been many cases of regulations introduced to control waste effluent flowing from chemical plants into rivers and other sources of drinking water. Other problematic issues such as the widespread release of chlorofluorocarbons (also known as CFCs) and other ozone layer-depleting chemicals into the atmosphere, resulting in global warming, arose from a period of industrial expansion, when the environmental effects of chemicals were either unknown or ignored, if considered at all.
However, despite the environmental disasters of the past, change is upon us. The movement is to all things green, and we can look to organic chemistry for more detailed solutions. Green chemistry can be defined in many ways: the phrase “benign by design” sums up its main aim very successfully. Practically speaking, green chemistry is essentially the treatment of waste from chemical reactions by preventative means. Consider preventative medicine – it takes no great exertion of the mind to realise that it is easier to undertake steps to prevent a disease than the disease is to treat when it takes hold, especially if a large number of people are at risk of developing the disease. Green chemistry is the same kind of idea – instead of spending money on destroying or cleaning up waste at the end of a process, redesign the process so that the waste is not an issue (or at the very least, less of an issue than it was before).
There are several ways to go about such a synthetic redesign. In their book Green Chemistry Theory and Practice, published in 1998, Paul Anastas and John Warner outline six main points to consider when transforming a chemical reaction to one that is more eco-friendly. It is recommended that before committing to a manufacturing process and setting it in motion, alternative feedstocks, reagents (reacting agents), solvents (a medium suitable to allow reactions to take place in) and catalysts should all be organic (or inorganic, as the case may be) food for thought (or re-thought) 1. Ideally, reactions should be monitored in real time, and the conditions altered in order to adhere to the production of products which are as environmentally-friendly as possible. The redesign process may even go so far as to assessing whether or not you really want to make the particular target molecule that you were initially set upon, depending on the compound’s environmental pros to cons ratio, amongst other reasons.
Five years before Anastas and Warner published their twelve principles of green chemistry in textbook form 2, Roger Sheldon published a paper on another equally green-revolutionary concept – the environmental factor. The environmental (or E-) factor is defined as the ratio of the mass of waste or by-product per unit of desired product from a chemical process 3. Many factors are considered when calculating the E-factor – for example, product yield, volume of solvents and reagents used and even fuel consumed (although quantifying this can occasionally be difficult) are all taken into account 4. Sheldon calculated typical E-factors for different chemical industries, noting that pharmaceutical companies had particularly high E-factor values – higher than oil refining, bulk chemicals and fine chemicals industries 5. The ideal value of the E-factor is zero, although from the numbers shown in the table below, the chemical industry still has quite a way to go yet before this ideal could ever be realised.
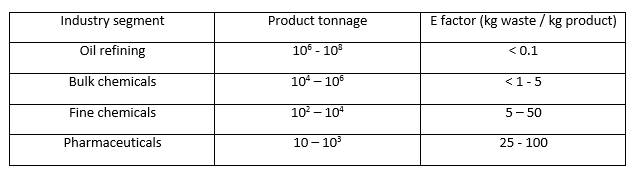
Table 1: E factors in the chemical industry. Image credit: Image created by the author, using data from R. A. Sheldon’s paper: ‘The E-factor: fifteen years on’, 2007, Green Chem., 9, 1273-1283.
One such way to achieve low (if not zero) levels of waste is to think atom economically. This term was introduced in 1995 by Barry Trost, when he published on the subject of catalysis 6. For a reaction of the type A + B + C → D + E, where D is the desired product, the atom economy is defined as the molecular weight of product D multiplied by 100 and divided by the sum of the molecular weights of A, B, C and E. In order for a chemical reaction to be considered as atom economical or efficient, all or most of the atoms present in the starting material(s) should be present in the product at the end of the reaction. As Sheldon noted in his 2007 paper “The E-factor: fifteen years on”, one chemical reaction which comes very close to the ideal is the Diels-Alder reaction. The Diels-Alder reaction is the process by which a diene (a molecule with two carbon-carbon double bonds in a set geometry) and a dienophile react together to form a cyclic organic molecule (see Figure 1 below for an example). Diels-Alder reactions occasionally require a class of compounds known as Lewis acids to accelerate the reaction. These Lewis acids are the only other material involved in the reaction which are not starting materials or solvent. As such, if Lewis acids were not used, the remaining solvent would be the only source of waste from the reaction, assuming 100 % conversion of the starting materials to products. It is also possible for the solvent to be distilled and then recycled.
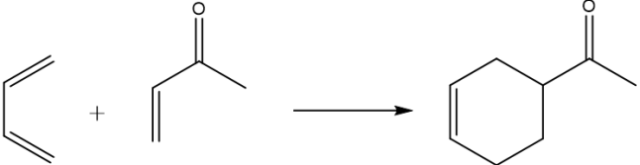
Figure 1: A typical Diels-Alder reaction. The diene is shown on the far left of the arrow and the dienophile on the left of the arrow. Image credit: Debbie Nicol. Image created using ChemBioDraw Ultra 14.0.
However, not all reactions are capable of this level of efficiency – not without a little extra help, anyway. In order to boost reaction rates (or even to get the reaction to go at all), catalysts can be introduced into the reaction mixture. A catalytic process involves the increase of reaction rate between two molecules, substrates or chemicals by a so-called catalyst, which itself is not consumed in the reaction. In fact, catalytic processes see the regeneration of the catalyst – in effect, it is recycled and thus can be re-used in later reactions. Whilst this is a good enough reason in itself to use a catalyst, another advantage of utilising a catalyst in a chemical reaction is that a significantly low amount of catalyst can be used with respect to the starting materials. Some reactions, such as a typical amidation (amide bond forming) reaction, require the use of additives or reagents in a stoichiometric amount i.e. one equivalent of additive/reagent to one equivalent of starting material. When catalytic activity depletes and the catalyst can no longer be recycled, the amount of waste produced is thus smaller than the amount of waste produced by non-recyclable stoichiometric reagents.
Whilst taking a green chemistry approach to creating a new chemical process seems easy enough, it can be a little more difficult to make pre-existing processes greener in nature. Available equipment can pose a serious limitation to what chemistry you can do as well as how you do it. An entire process redesign, if it involves the replacement of some equipment or refurbishment of a whole chemical plant, can be a monetary drain. As well as refurbishment costs, such an undertaking could also affect or halt the production of a particular chemical or medicine. The longer that no product is being manufactured, the more money that is lost in the pursuit of an environmentally-friendly method of chemical production. This may deter companies from implementing (or even attempting to implement) such changes to their manufacturing processes. Of course, introducing such a process to reduce waste production would mean serious savings in terms of the amount of money previously spent on waste remediation. Process redesigns could also see savings in material costs. For example, in 2002, Pfizer, a world-leading pharmaceutical company, received a Presidential Green Chemistry Award in the category of Greener Synthetic Pathways for their successful redesign of the process used to produce sertraline, the active ingredient in their anti-depressant Zoloft. Amongst the numerous tweaks to the process was the replacement of four different solvents with a single solvent. This meant that it was no longer necessary to purchase, use, distill and recover four solvents at the end of the process – only one. The new process also allowed Pfizer to double the yield of active ingredient being produced.
For those companies in the chemical industry that are not excited by the prospect of a process overhaul or the inspiring synthetic challenges green chemistry allows, legislation introduced in 2007 by the European Union entitled REACH (Registration, Evaluation, Authorisation and restriction of CHemicals) could see a change in feeling on the subject. One of the principal aims of REACH is to protect the environment and human health from unnecessary chemical pollution or exposure to hazardous chemicals 7. The legislation requires that any substance produced by manufacturers based in the EU in excess of 1 tonne per year must be registered with the European Chemicals Agency (ECHA), in order that risk and hazard assessments on these substances can be carried out to determine risk to the environment and to human health. Failure for a company to do so means that they cannot produce or sell their chemical products legally, and penalties for non-compliance in extreme cases include unlimited fines and up to two years’ imprisonment 8. REACH is also capable of banning the use or supply of certain substances if the risk they pose is great enough (for example, if they are carcinogenic), and as such has control over materials such as solvents that the pharmaceutical industry can use in the synthesis of the active ingredients of their drugs. The use of chemicals on a large scale is as much of a concern for policy makers as it is for process safety chemists and the chemical industry as a whole.
It is worth noting that green chemistry is not limited simply to the prevention of waste – the use of sustainable materials is another area of research enthusiastically promoted by the principles of green chemistry. For example, biofuels are an attractive, recyclable energy source or feedstock compared to crude oil or petroleum-based fuels. The term ‘sustainable’ also applies to catalysts. Many catalytic processes involve palladium-centric catalysts. Whilst having the disadvantage of being toxic, elemental palladium is also not particularly abundant in nature, leading to great expenses for these types of catalysts. There has been a great deal of research into replacing palladium-based catalysts with nickel-based catalysts. Nickel and palladium share similar electronic features (being in the same group of the periodic table and thus having an identical outer electron configuration) and express similar catalytic cycles. Nickel has the advantage of being far more abundant in the earth’s crust than palladium, and as such it would be significantly more cost-effective to introduce nickel-catalysed reactions into a pharmaceutical process, for example. Iron catalysis has also attracted a lot of research attention in recent years.
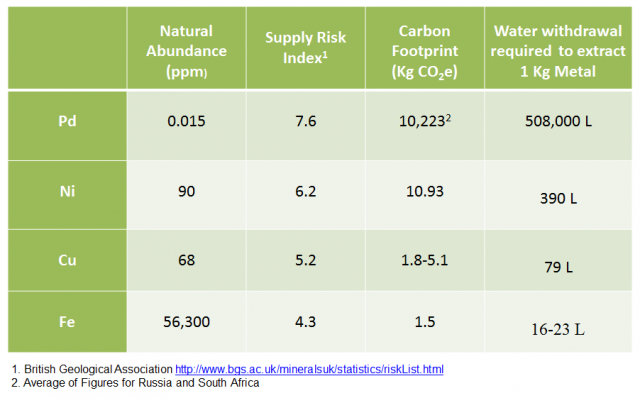
Figure 2: Table of natural abundances and other sustainability metrics of selected metals with catalytic roles or catalytic potential. Image credit: Taken from a presentation entitled ‘Green Chemistry, Sustainable Catalysis and Environmental Assessment’ by Peter Dunn (presented at the SCI Meeting, London on 11/11/2014) and published here with full permissions. Sources of figures included in the image.
The above is only a glimpse of the wide range of green alternatives to current manufacturing processes. As well as obvious cost savings, green chemistry also offers the chance to be increasingly innovative with organic synthesis – why should we stick to outdated, unimaginative and hindering processes? Get your thinking caps on, chemists. Let’s save the environment, and let’s do it with some style.
With thanks to Peter Dunn.
Specialist edited by Emilie Steinmark and copy edited by Charlie Stamenova.
References
- Paul T. Anastas and John C. Warner, ‘Green Chemistry Theory and Practice’, Oxford University Press Inc., New York, 2000 (paperback)
- The 12 Principles of Green Chemistry, Sheldon.nl.
- Green chemistry metrics entry, Wikipedia.
- Roger A. Sheldon, ‘The E-factor: fifteen years on’, 2007, Green Chem., 9, 1273-1283
- United States Environmental Protection Agency website.
- B. M. Trost, ‘Atom Economy – A Challenge for Organic Synthesis: Homogeneous Catalysis Leads the Way’, 1995, Angew. Chem. Int. Ed., 34, 259-281
- Health and Safety Executive website.
- Health and Safety Executive website.