Observing the cosmos from deep within Antarctic Ice
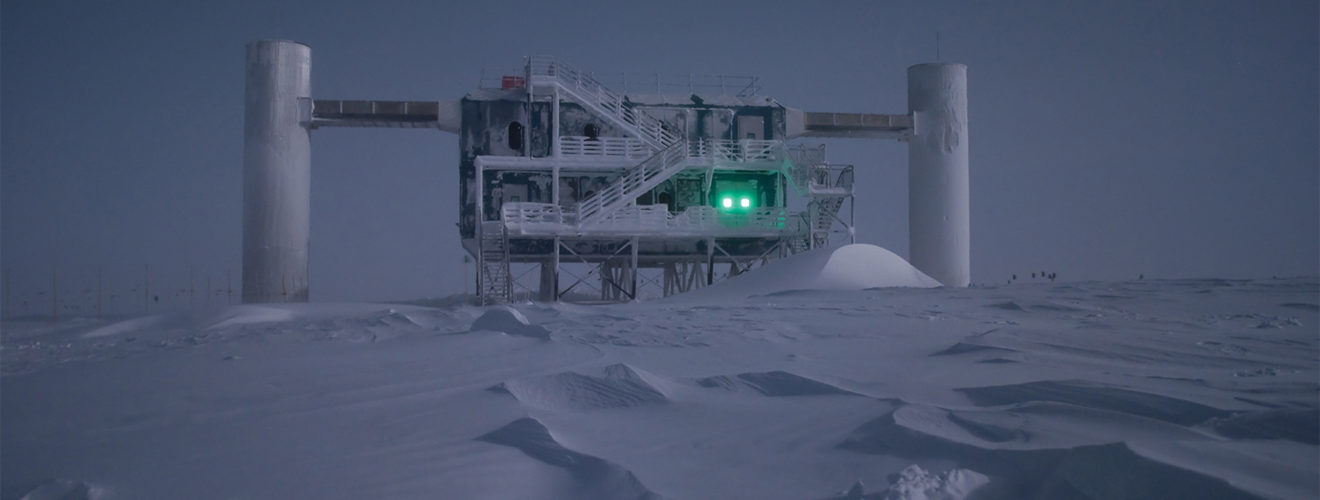
Buried roughly a mile deep into the Antarctic ice sheet, the IceCube Neutrino Observatory serves as a deep-space telescope, allowing scientists to see distant cosmic events by detecting the neutrinos they generate.
These high-energy “ghostly” particles travel at nearly the speed of light from the sun, from within the Milky Way, and from outside the galaxy. Neutrinos are the second most ubiquitous particles (comprising 25% of fundamental particles), second only to photons left over from the Big Bang. Yet, because they barely interact with other matter, they are arguably the least understood.
The polar ice sheet in the Antarctic supplies the main ingredient needed for a neutrino detector: a large space that’s totally dark and transparent. IceCube’s sensors, known as digital optical modules (DOMs) detect neutrinos indirectly by spotting evidence of muons, secondary particles produced when a single neutrino crashes into a proton or neutron inside an atom. As it propels through the ice at a speed faster than light travels through the ice, a muon produces a trail of blue light—Cherenkov radiation—that acts like an arrow pointing back in the direction of the neutrino’s origin.[1]
Since neutrinos move in a straight path, without being deflected by magnetic fields or absorbed by matter, scientists can trace them back to their origins—some of the most violent and least understood objects and events in the universe, like the explosive stellar death of a star (a supernova), active galactic nuclei or gamma-ray bursts. Current theory suggests that such violent phenomena, along with cosmic rays, accelerate neutrinos to incredible energies.
Cosmic rays, consisting of the nuclei of hydrogen, helium and other elements that travel through space, are easier to detect because they are larger and carry an electrical charge. Yet, they are easily deflected by magnetic fields that erase evidence of their origins. Neutrinos, on the other hand, are rarely absorbed by matter and are not deflected by magnetic fields, thanks to their neutral charge, so they preserve information about the location and energy of the events that produced them. They are, therefore, excellent astronomical messengers of information about the objects or events in which they originate.[2] They can provide accurate information about where they came from in the distant universe, even billions of light-years away.
Back in 2017, for example, IceCube detected a neutrino that, for the first time, scientists were able to trace back to its cosmic source in the constellation Orion: a flaring black hole the size of a billion suns, 3.7 billion light-years from Earth, known as blazar TXS 0506+056.[3] Blazars are a class of active galaxy nuclei (AGNs) that form from a supermassive black hole, and although the black hole devours most of the material around it, some of the gas and dust is deflected into powerful jets which accelerate matter, creating neutrinos and some cosmic rays.[4]
Scientists are now looking to expand the capabilities of IceCube with the new and improved IceCube-Gen2 at the South Pole (set to launch in 2033). It will increase the annual rate of observed cosmic neutrinos by a factor of ten compared to IceCube and will be able to detect cosmic sources five times fainter than its predecessor.[5] IceCube-Gen2 will also extend the energy range of IceCube by several orders of magnitude, providing us with more windows into the “extreme universe.”
Edited by Liam Butler
Copy-edited by Claire Thomson
[1] https://www.earthmagazine.org/article/astronomy-under-ice-scientists-use-antarctic-ice-study-some-tiniest-particles-cosmos/
[2] https://icecube.wisc.edu/about-us/faq/
[3] https://theconversation.com/the-icecube-observatory-detects-neutrino-and-discovers-a-blazar-as-its-source-99720
[4] https://www.pnas.org/content/115/34/8463
[5] https://iopscience.iop.org/article/10.1088/1361-6471/abbd48/pdf